Put on your high-energy sensitive glasses! Go on now, don’t be shy. What do you see when you look out into the universe?
If (like me), you left that particular pair of glasses in your other lab coat, it is also perfectly fine to look at the all-sky map from the Fermi Space Telescope:
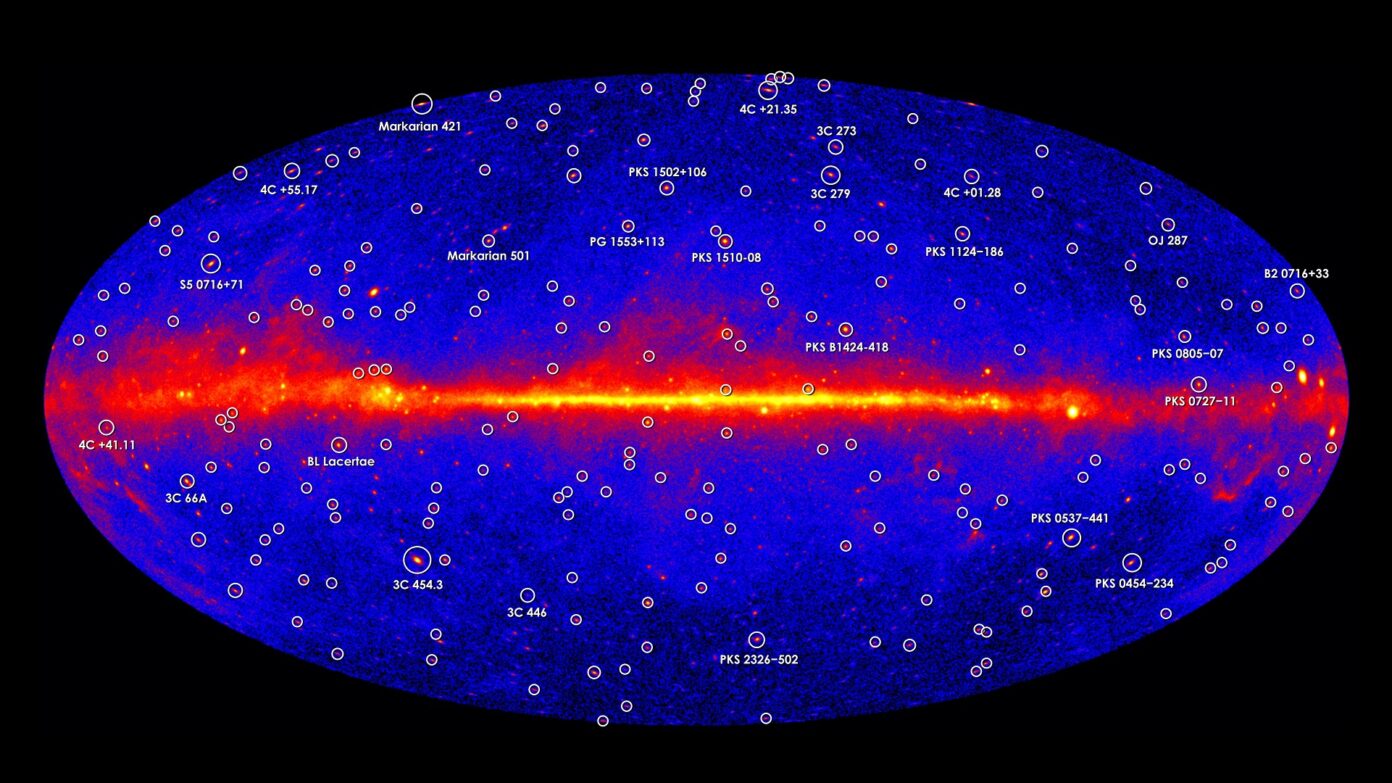
Image credit: NASA/DOE/Fermi LAT Collaboration.
The universe is filled with weird and exciting astrophysical sources: pulsars, active galaxies, supernova remnants, and more, located all around us. We routinely study these objects across all wavelengths of the electromagnetic spectrum, from radio waves to gamma-rays. Using both ground-based and space-based telescopes, we can obtain spatial information (imaging), timing information (variability) and spectral information (energy).
A property of the observed electromagnetic radiation that has historically been overlooked – at least in the high-energy range – is the polarimetric information. Roughly speaking, polarization can be thought of as the direction in which the waves of the radiation oscillate. Visible light can become polarized, for example, when reflected from a flat surface – this is the reason why you want to use polarizing sunglasses, to reduce glare from water surfaces, wet asphalt, snow, etc.
It turns out that high-energy radiation becomes polarized in much the same way, and the polarization can provide information about sources that are too distant and too compact to be imaged by traditional means. It is like looking up at the night sky with your bare eyes: you can see point-sources of light but you cannot resolve any structures. This is where polarization can swoop in to save the day, because polarization is sensitive to the ‘history’ of the radiation: to what has happened to the radiation before it reaches our telescopes. This can include magnetic fields encountered, layers of dust or gas where the radiation can become reflected, regions of strong gravity where effects of general relativity alter the radiation through the curvature of space-time, etc.
For sources bright in X-rays and gamma-rays, measuring polarization becomes an attractive prospect. But there is a catch! High-energy polarimetry is a very ‘photon-hungry’ business: whereas a source can be detected with as few as ~10 photon counts in a detector, and ~100 counts can be enough to determine spectral properties, a polarization measurement may require a hundred or a thousand times more data than that. As a result, high-energy polarimetry has had to take a back seat as compared to the more ‘low-hanging fruit’ offered by spectral and imaging measurements.
Astrophysical X-ray polarimetry kicked off in the 1970s with a successful measurement using the OSO-8 satellite. Despite the promising start, the field was soon to fall dormant. The Stellar X-ray Polarimeter was supposed to fly on a Russian mission in the early 1990s, but with the fall of the Soviet Union, the satellite was never launched. The 2000s saw a renewed interest in X-ray polarimetry, with measurements using detectors on the INTEGRAL satellite, but these instruments were not calibrated for polarization, so measurements were plagued by large systematic uncertainties. Only more recently are we seeing dedicated polarimeters, both balloon-borne like the PoGO mission and satellites like IXPE.
Now, the field could be up for a significant addition, as an international team with members from the United States, Sweden and Japan is just getting ready to launch XL-Calibur, a balloon-borne high-energy polarimeter. XL-Calibur is a so-called Compton telescope, based on the principle that for photons interacting in the detector, the characteristic scattering angles depend on the polarization of the incoming radiation (see figure 2).
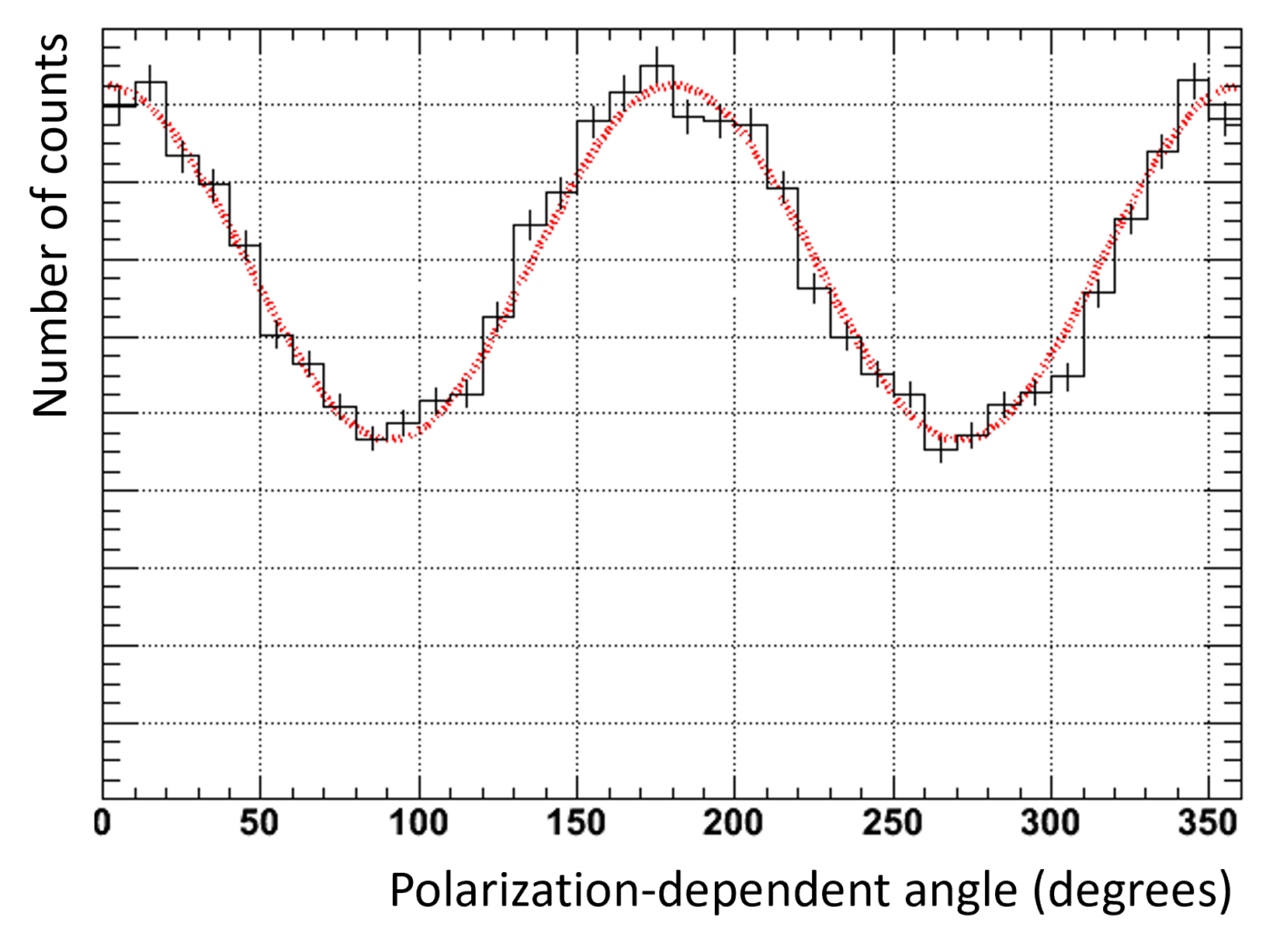
In order to collect X-rays from astronomical sources, an X-ray ‘lens’, or mirror, is used. Since X-rays really like to go through materials, the mirror can only focus/deflect the rays by the tiniest amount, no more than 1° in angle. The result is a telescope with a very long focal length: about 12 meters. The mirror is located on one side of a carbon-fiber structure, with the X-ray detector on the other side – see figure 3.
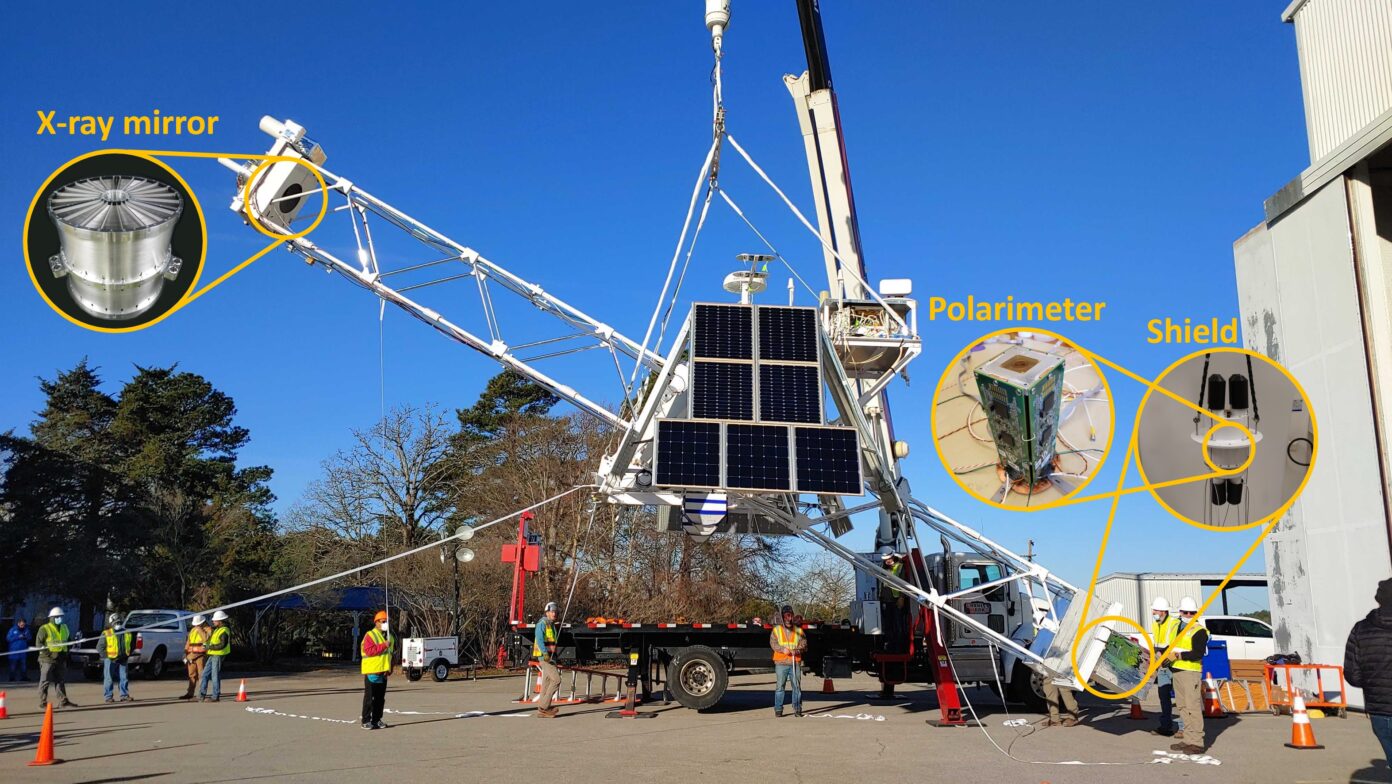
Since X-rays are absorbed in the Earth’s atmosphere, the instrument is launched on a stratospheric balloon, to an altitude of about 40 km. To reach so high, a balloon with a volume of more than one million cubic meters is required. Once afloat, the ‘WASP’ system (an acronym for Wallops Arc-Second Pointer) allows the telescope to acquire and track sources in the sky.
At the time of writing, the team is just getting ready to travel to the Esrange Space Center in northern Sweden. The launch window is scheduled to open on the 8th of May 2024. Following the release from the 50-tonne ‘Hercules’ launch vehicle (Figure 4), the balloon takes a few hours to reach float altitude, where it will drift with stratospheric winds in a westerly direction. After a week-long flight across the Atlantic, the instrument can land by parachute in Canada, to be recovered by ground teams and refurbished for subsequent flights. The termination of the flight, initiated by remotely detonating a pyrotechnic cutter, is carefully timed to minimize the risk of landing in any one of Canada’s thousands of lakes…
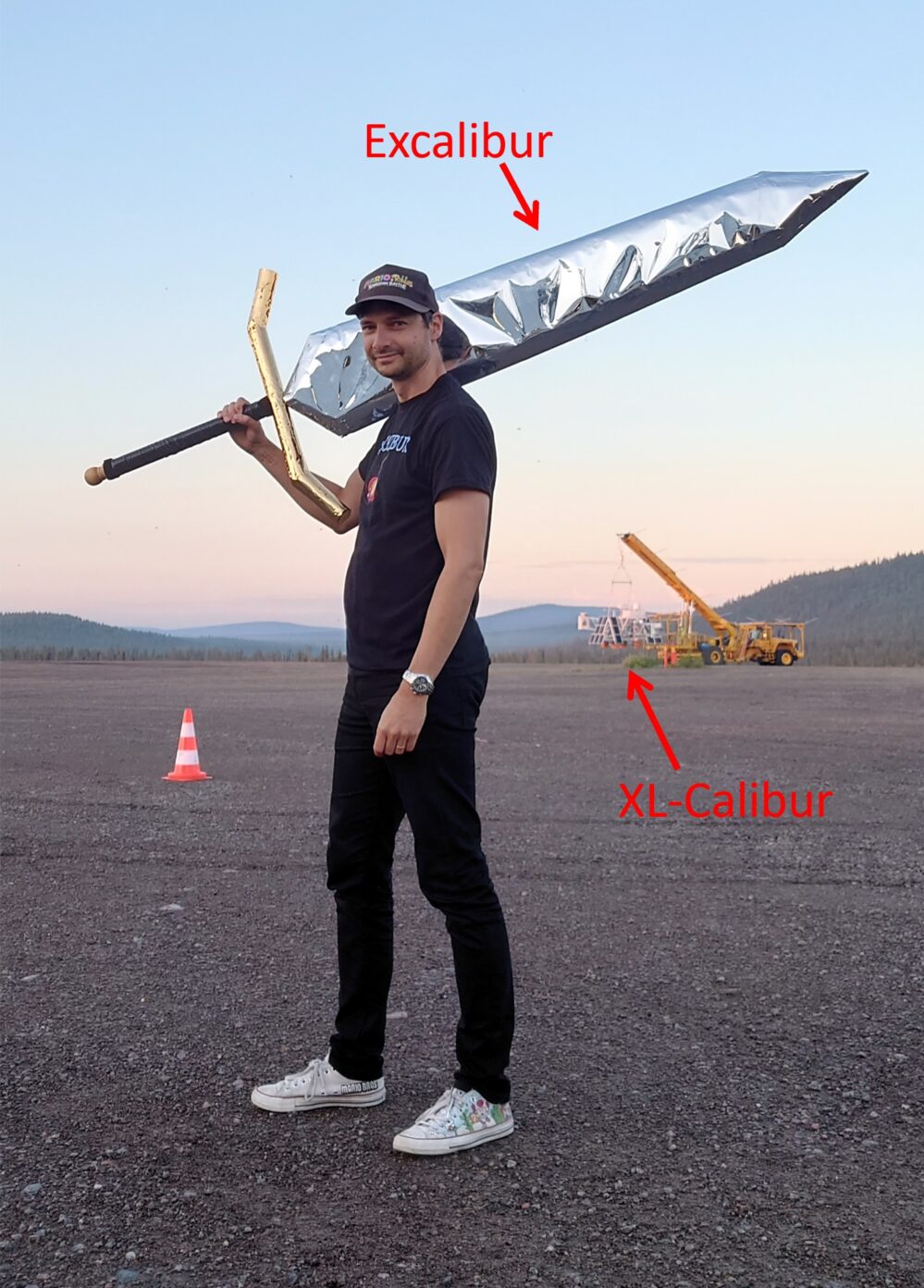
One of the main targets for XL-Calibur is the Crab system: a pulsar (a rapidly rotating neutron star) at the center of a nebula produced in the aftermath of a supernova that was observed by Chinese astronomers in 1054 AD. Neutron stars such as the one in the Crab system are truly fascinating. The mass of a pulsar is comparable to that of our sun, but it is compressed into a very small celestial body: perhaps only about 10 kilometers across. This leads to unfathomably high densities (a ‘teaspoon of neutron star’ weighs about as much as the Himalayan mountain range) and absurdly strong magnetic fields (up to a trillion times stronger than the Earth’s magnetic field). Such extreme conditions can never be recreated on Earth (maybe just as well) and observing these astrophysical sources can thus help us understand how matter behaves in extreme environments.
Although the Crab system is one of the most-studied X-ray objects in the sky, we still do not fully understand how the high-energy radiation is produced near its pulsar. Polarization measurements can break degeneracies between different proposed radiation models, so XL-Calibur is expected to shed light (pun intended) on these fascinating sources. Fingers crossed for a successful launch in just a few weeks from the time of writing!