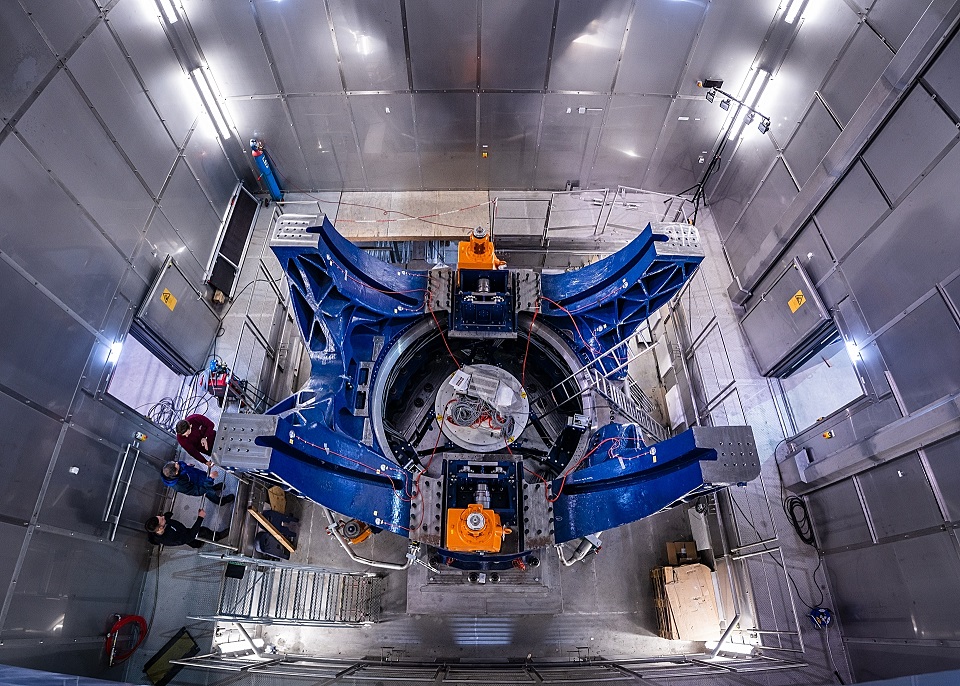
From science to society: why research facilities like DRESDYN matter for our future
Introduction
Large scale research facilities form a key component of modern scientific research. Large amounts of money and many resources are invested in such facilities. One such facility is DRESDYN (the DRESden Sodium facility for DYNamo and thermohydraulic studies), currently being constructed on the grounds of the Helmholtz Zentrum Dresden-Rossendorf in Germany. The DRESDYN experimental facility is the world’s first large-scale facility designed to better understand the behavior of magnetic fields and fluids undergoing motion. It hosts two main experiments, one to better understand the Earth’s precession and dynamo, and one to learn more about magnetic and fluid instabilities.
The first experiment
The first experiment is a precession driven dynamo experiment – see figure 3. Imagine a top spinning. As it spins it is slightly tilted and it wobbles, drawing an imaginary circular path with its axis. Just like the top, Earth too is spinning on a tilted axis and due to the Sun’s gravitational pull, it wobbles. This results in a motion that we call precession. Next, think of a bicycle dynamo which generates electricity by converting mechanical energy into electrical energy as you pedal. Similarly, a magnetic dynamo generates a magnetic field by converting kinetic energy from the motion of charged particles in a conducting material such as a liquid metal or plasma, into magnetic energy. In the case of this experiment, the liquid metal would be sodium. The concept of a magnetic dynamo is important for the magnetic fields of stars, as well as for the Earth’s magnetic field, which is generated by the movement of molten iron in its core.
If we now combine these two ideas, the precession in the experiment will drive motions in the liquid sodium inside it, which will then in turn drive a dynamo generating and maintaining a strong magnetic field.
This experiment is mainly designed to help us better understand the role of the Earth’s dynamo and its precession. It is about understanding how the Earth’s magnetic field works and how it is affected by changes in the Earth’s orbit. Scientists have long thought that changes in the Earth’s orbit could be a source of energy for its magnetic field.
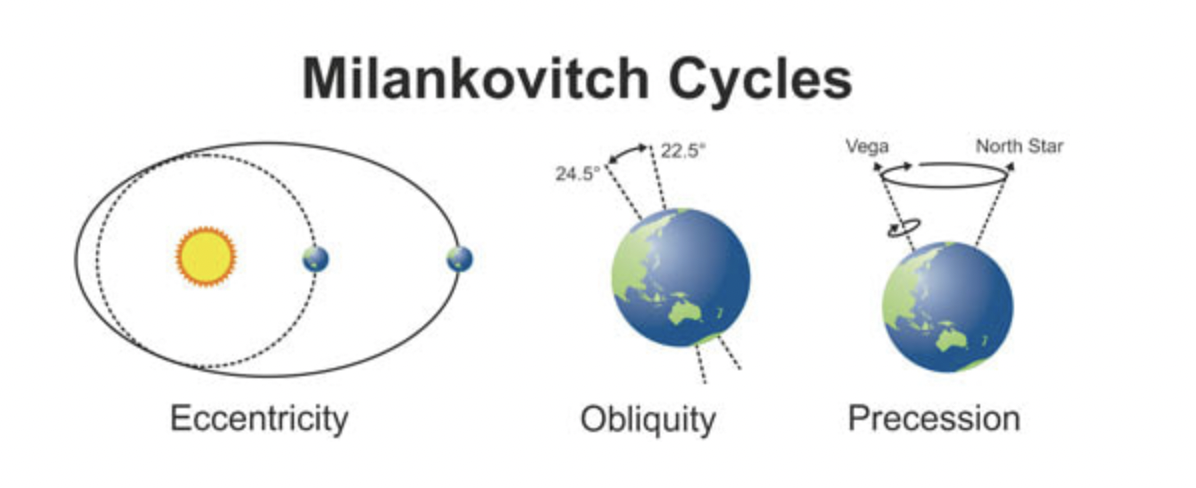
We can study how the strength of the magnetic field changes over time by looking at the so-called Milankovitch cycles (see figure 2): changes in the Earth’s orbit that happen over thousands of years. Milankovitch cycles essentially show the combined effects of changes in the Earth’s motions on our climate. These changes include how circular or elliptical the orbit is (its eccentricity), how the Earth wobbles on its axis (its precession), and how tilted it is (its obliquity). They can also affect how often the magnetic field flips direction.
Researchers have already shown that there is likely some connection between the Earth’s magnetic field changing, and variations in our climate in the last 5000 years. This might also imply that the magnetic field has an impact on the ice age cycles. However, there is still a lot we don’t know about what causes the changes in the Earth’s orbit.
The researchers at DRESDYN will explore how dynamos could be generated and what effects changing them may have. One idea is that the Earth’s precession may have helped create the magnetic field that protects us from harmful cosmic radiation which would make life on Earth impossible. Furthermore, it will be interesting to better understand the significance of the size of the Earth’s axial tilt (23°).
What will this first experiment look like? Its main component will be a large cylindrical vessel that will be filled with sodium. The cylinder spins 10 times per second along its central axis, and once per second along an axis whose inclination can be varied between 45° and 90° to the cylinder. These motions are so extreme that to withstand the stresses, the set-up had to be mounted on an extremely massive specially built base, itself mounted on several columns buried more than twenty meters deep into the ground.
Unfortunately, sodium reacts extremely when in contact with air or water. This means that it can catch fire and burn. Therefore, the experiment is contained in a large box which can quickly be flooded with Argon (which pushes air out of the room and does not react strongly with anything) in the case of any sodium leak.
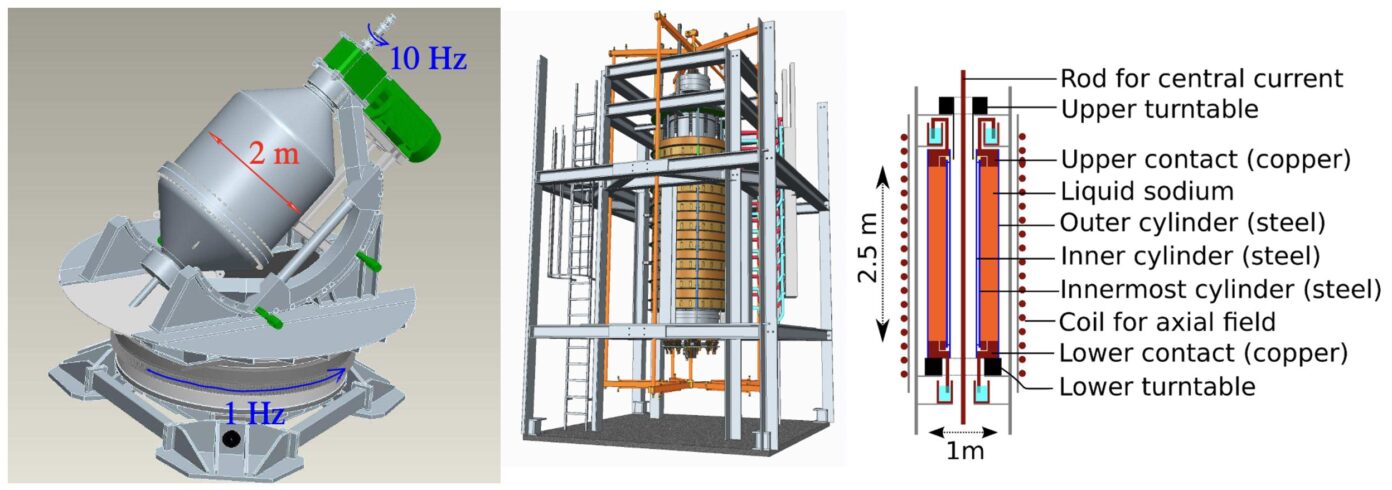
The second experiment
The second experiment at DRESDYN will investigate two types of instability relevant to many astrophysical phenomena: the Magneto-Rotational instability (MRI) and the Taylor instability (TI).
To better understand what we mean by an unstable liquid, imagine a pot of water on a stove. If you slowly heat up the water, it will eventually start to boil and create small bubbles. In this case the water is still stable, because the bubbles are small and don’t significantly affect the motion of the water. However, if you suddenly turn up the heat there will be more and larger bubbles, causing the water to start boiling violently and spilling out of the pot, i.e. the water becomes unstable. This instability arises because a small change (in this case, the sudden increase in heat) caused a large-scale and unpredictable change in the water’s motion and behavior. In the case of the MRI experiment, the researchers are interested in studying how magnetic fields can make fluids unstable. The Magneto-Rotational instability happens when a weak magnetic field makes a spinning liquid unstable. This can cause the liquid to become turbulent and create swirls.
Now imagine the pot of water from before again. As you turn up the heat, a sort of wavy pattern forms on the water’s surface. The hills and valleys form because of an interplay of fluid flow and gravity: the Taylor instability. These patterns grow stronger with time and eventually small whirlpools or turbulences can occur. Thus, the Taylor instability happens when two liquids with different densities are rotating together, usually driven by a current applied to the liquids. The two liquids in this case can be compared to a liquid metal battery: these are made up of a layer of a metal alloy and one of metal, separated by a thin layer of molten salt. As currents are applied and the fluids rotate against each other patterns can form, which can lead to more turbulence and the formation of an instability.
The second experiment at DRESDYN aims to investigate different types and combinations of Magneto-Rotational and Taylor instabilities – see figure 3 on the right. A coil producing a strong vertical magnetic field is wrapped around the central, sodium filled cylindrical vessel in this experiment. This cylinder is mounted between two turntables that can spin it around its central axis.
Industrial Benefits
Next to the astronomical and geo-physical research conducted at the facility, DRESDYN will also conduct experiments with implications for the energy production industry. Understanding how liquid metals behave in the vicinity of different magnetic fields is crucial in being able to produce better large-scale liquid metal batteries for renewable sources such as solar and wind plants. Currently, these batteries still have a tendency to self-destruct. In other words, these batteries will, under certain conditions, experience performance losses or internal damage which may lead to leaks or even explosions. This poses a risk to the battery and its surroundings.
The energy gained from these renewable sources fluctuates greatly, which makes it difficult to store, especially in larger batteries. Smaller versions of such batteries have already been considered, but we generally want to build bigger ones for more efficient storage and more stability in the electricity grid. As the size of the battery increases, however, instabilities such as the TI may become relevant and pose a serious issue.
So what does this mean…?
An argument often made against investing in large-scale research facilities such as DRESDYN is that their benefits are not always immediately apparent. However, it is important to remember that many of the scientific breakthroughs that have transformed our world, such as the development of antibiotics, GPS and the invention of the internet, were the result of decades of scientific research.
In the summer of 2022 I worked at the Helmholtz-Zentrum Dresden-Rossendorf (HZDR) myself and met many of the researchers that will be involved at DRESDYN. I got to look at the parts of the facility that had been constructed by then, and was inspired by my colleagues’ fascination for this experiment. Despite the fact that they faced many challenges in gaining the support for this project from the general public, politicians and investors, their enthusiasm about the upcoming facility was evident and I was very thrilled to learn about the forefront of current research.
This excitement pertains not only to the scientific advances this experiment will undoubtedly yield, but also to the potential impacts this could have on Dresden-Rossendorf as a whole. Such large-scale facilities require a vast array of skilled professionals, from physicists and engineers to technicians and support staff. They provide a unique opportunity for students and early-career researchers to gain hands-on experience working on cutting-edge projects alongside some of the brightest minds in the field. This exposure to scientific research can ignite a passion for discovery and innovation in young people, inspiring them to pursue careers in science, engineering, and technology.
Conclusion
Overall, the DRESDYN facility will help us gain important insights for astrophysical phenomena such as the behavior of the Earth’s magnetic field and accretion disks around astrophysical objects. It will also yield results for more industrial purposes such as investigating the potential difficulties that large-scale liquid metal batteries face. However, DRESDYN is also a testament to the value of large-scale scientific research facilities. These facilities require significant investments of time, money, and resources, but they are necessary for advancing our understanding of fundamental science, engineering, and technology. In other words, these facilities provide a unique opportunity to study complex phenomena that cannot be easily reproduced in a traditional laboratory setting, while advancing our understanding of fundamental science and technology, creating jobs, and inspiring the next generation of scientists and researchers. As such, I believe that investing in large-scale research facilities is a worthwhile use of resources and can help drive scientific progress and innovation for years to come. I am looking forward to seeing the fascinating science that will be conducted at the DRESDYN facility over the next years!
Sources
Stefani, F., Eckert, S., Gerbeth, G., Giesecke, A., Gundrum, Th., Steglich, C., Weier, T., & Wustmann, B. 2012, arXiv e-prints, arXiv:1201.5737, physics.flu-dyn
Weckbrodt, H. 2018, Oiger, https://oiger.de/2018/07/30/kuenstlicher-erdkern-startet-erst-2020-in-rossendorf/168859
Nachrichten, Dresdner Neueste. Künstlicher Erdkern startet erst 2020 in Rossendorf. DNN. https://www.dnn.de/lokales/dresden/kuenstlicher-erdkern-startet-erst-2020-in-rossendorf-B3XPRBKQCFJGZ37K774M5YA6IE.html
TU Dresden. 2017, Sep. Dresden sodium facility for Dynamo and Thermohydraulic Studies, https://tu-dresden.de/ing/maschinenwesen/ifkm/dmt/forschung/projekte/DRESDYN
SBS Bühnentechnik. Dresdyn, https://www.sbs-buehnentechnik.de/index.php/de/forschungstechnik-dresdyn